In Arctic regions, perennially frozen ground, i.e. permafrost, contains twice as much carbon as currently stored in the atmosphere. With the warming around the globe, especially in the polar regions, permafrost is thawing with the potential of releasing up to 195 Pg of carbon by 2100. When released in the atmosphere, carbon as CO2 and CH4 enhances the increase in air temperature and thereby permafrost thaw. This positive feedback between permafrost thaw, carbon release and increase in air temperature is called the permafrost-carbon feedback.
Thawing of Yedoma (i.e. ice-rich permafrost, fig.1) deposits leads to massive landscape modifications. The melting of ice-wedge networks and other excess ice usually leads to the collapse of the soil surface followed by thermokarst lake formation (fig.1). With time, the lake can extend horizontally and vertically and eventually create a drainage pathway. Upon lake drainage, sediment deposits remain within the dried basins, which are called “Alas” containing ice wedges which can once again thaw. At the Arctic scale, lake and drained lake basin systems can represent up to about 50% of the circumpolar Northern Hemisphere permafrost region lowland areas.
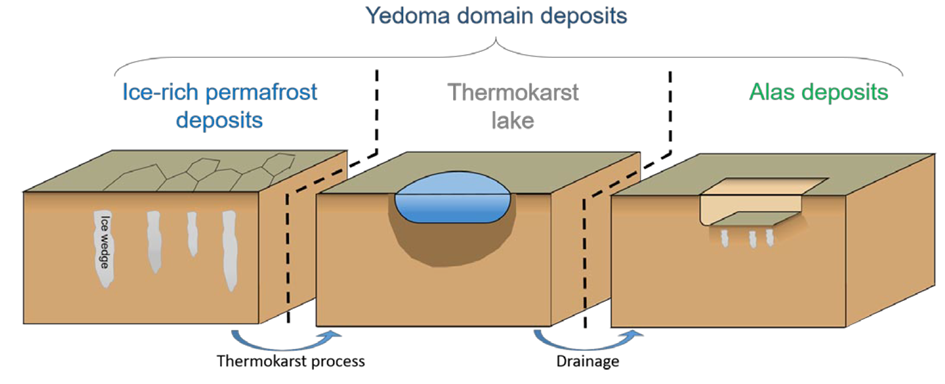
Figure 1: Schematic view of Yedoma and Alas (drained thermokarst lake basins) deposits considered for mineral element stock calculations.
With this ice-rich permafrost thaw, the carbon once trapped in the soil becomes available for microbial decomposition potentially releasing huge amounts of carbon in the atmosphere. But could it exist, natural buffers in soils that have the power to protect this newly thawed carbon from being emitted as greenhouse gases? Yes! Mineral elements such as iron (Fe), manganese (Mn), aluminum (Al) and calcium (Ca) are able to protect physically (by the formation of aggregates) as well as physico-chemically (through complexation and association) this organic carbon from being decomposed by micro-organisms in the soils. However, it is not said that upon permafrost thaw, these interactions will hold. Indeed, the thawing and draining of ice-rich deposits triggers shifts in soil or sediment properties, such as the redox potential or pH values. These shifts drive changes in biogeochemical processes with potential consequences for mineral–organic carbon interactions and thus decomposition rates. The aim of this paper is to assess the fate of these mineral-organic carbon interactions during the different stages of the thermokarst process and thereby the fate of this natural buffer.
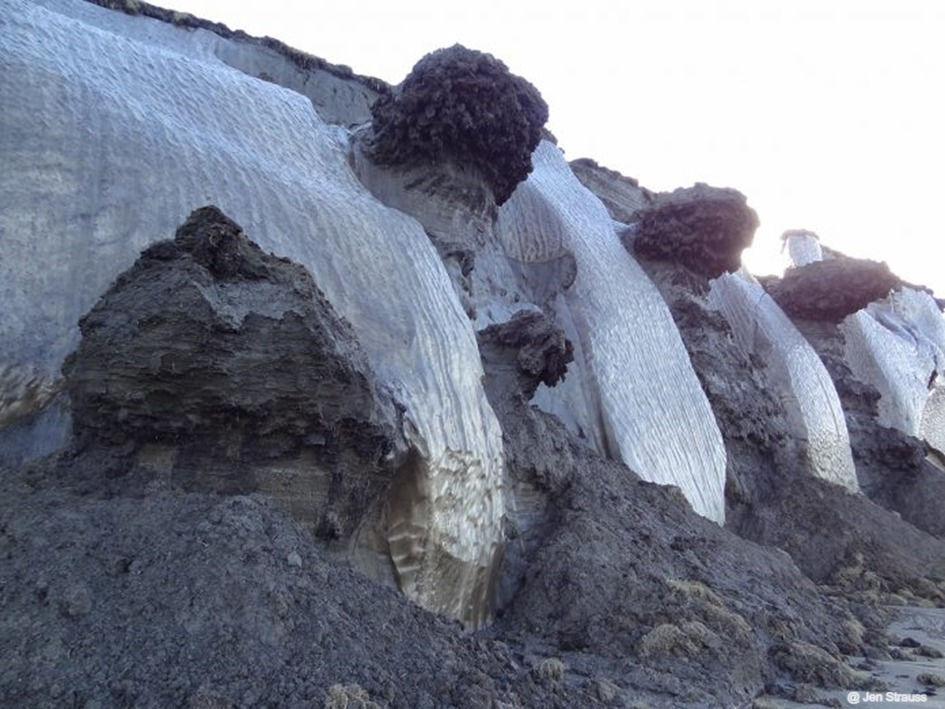
Figure 2: Ice-rich permafrost deposits formed thousands of years ago. Credits: Jen Strauss.
The results show that the state of the minerals-organic carbon interactions is dynamic along the time sequence. Upon thermokarst lake formation these interactions (complexed organic carbon and associated to mineral surfaces) are decreasing creating a window of time during which organic carbon is more vulnerable to mineralization but increase again in the Alas deposits, i.e. once the thermokarst lakes disappear and refreeze protecting organic carbon again. Overall, we found an increase in the supply of stabilizing Fe, Mn, and Al surfaces and Fe, Mn, Al, and Ca cations, providing an increased protection for organic carbon against mineralization. We also found an increase in metal to carbon bindings within organo-metallic complexes on thermokarst lake drainage and refreezing again showing an increased protection. All these contributed to the concomitant decrease in CO2 and CH4 emissions on secondary thawing of Alas deposits.
To read the full paper: https://onlinelibrary.wiley.com/doi/abs/10.1002/ppp.2162
Written by Eléonore Du Bois d'Aische
Comments